JWST + NIRSpec Cookbook
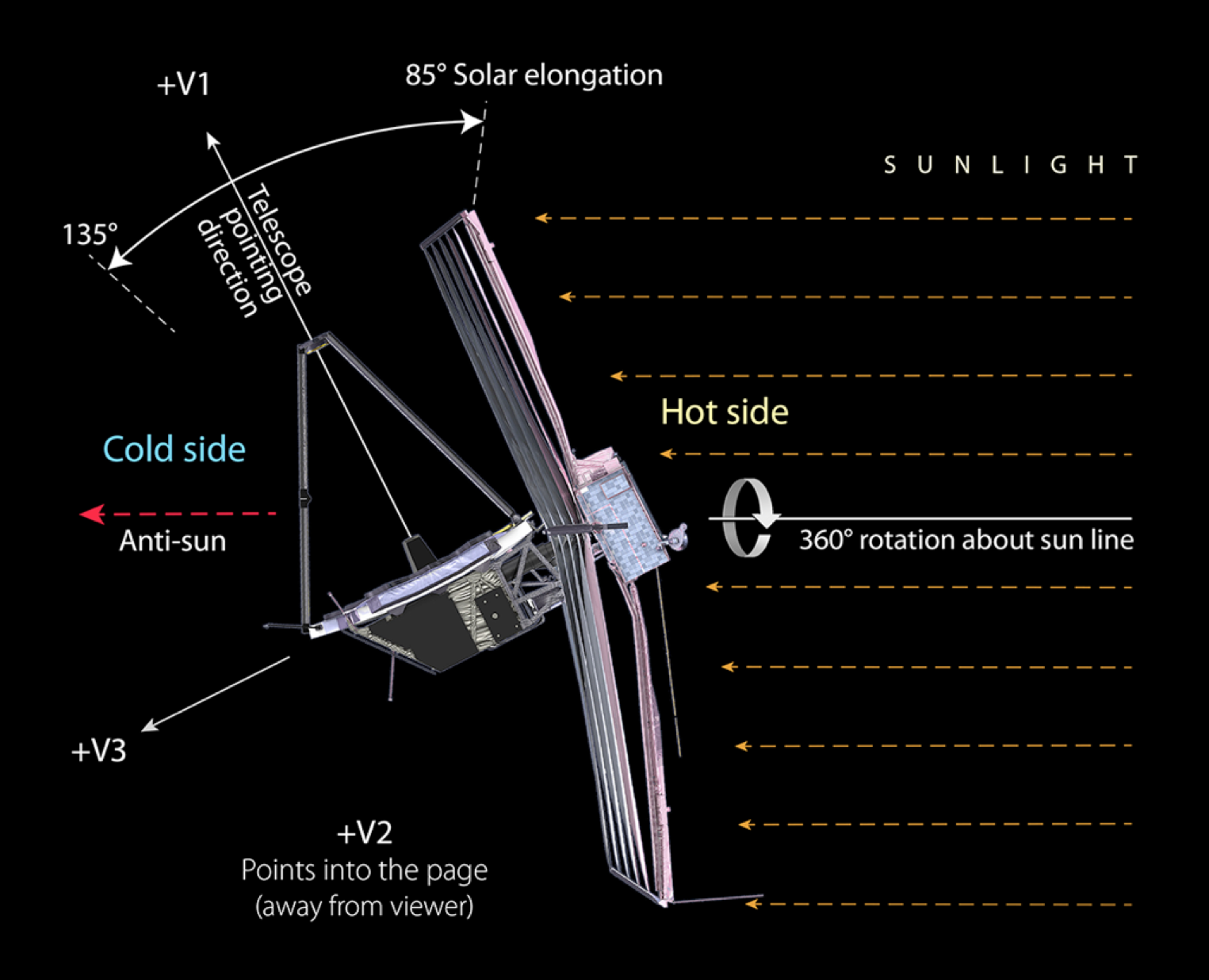
As the dealine for Cycle 1 General Observations is approaching (2018 April 6th – see here), I have put up a cookbook specific to solar system observations with NIRSpec. Owing to the GTO and ERS proposals, I have been through the process of using the ETC (exposure time calculator) and APT (astronomer proposal tool) extensively (albeit for NIRSpec), and I know about the learning curve… If you have a good science idea, this might actually take quite some time to translate it into a JWST proposal.
In this cookbook (download PDF – upcoming) or the related pages I have been putting up (here), you will find some generic information on NIRSpec, JWST features relevant to moving targets, and quick guides to the ETC and APT. However, please note that the official STSci/NASA webpages should always be considered as your bible – be sure to actually check them, as up-to-date information will always be found there first. I only mean to provide you with a basic guideline to get you started on your proposal.
Active Centaurs
The need for a particular figure in the review paper that the ISSI team is writing on Main Belt Comets was for me the occasion to get back to my work on active Centaurs. I thought I’d say a word about that here… Centaurs are a population of icy bodies which are orbiting the Sun roughly between Jupiter and Neptune. Initially, they were stored in the transneptunian region, on excentric orbits that got them close to Neptune from time to time. Eventually, their orbits got destabilized by such close encounters, and they ended up on unstable orbits in the giant planet region. Ultimately, the fate of most Centaurs is to get ejected from the Solar System. The others will become Jupiter Family Comets (JFCs), like the notorious target of the Rosetta mission, 67P. Dynamical studies show that some JFCs can go back to the giant planet region, and this ping-pong “game” can be played for about a dozen time.
One interesting feature of the Centaur population is that about 10 to 20% of them are active, loosing mass like comets do. However, the driver for the typical comet activity is sublimation, especially sublimation of water ice when comet nuclei get closer than 3 au of the Sun. In the giant planet region, water ice is thermodynamically stable: it does not sublimate, and cannot drive the activity of Centaurs. While trying to identify the source of Centaurs activity, Jewitt (2009) noticed that the physical and orbital properties of active Centaurs are such that their activity should be thermally driven, hence a phase transition like the crystallization of amorphous water ice, which releases trapped volatile molecules in the process, could actually fit the data. So back in 2012, I studied the survival of amorphous water ice in Centaurs, and how crystallization could contribute to their activity (article here). I performed the study so it would have a statistical significance, so no real Centaur is modeled here, but any real Centaur has its place in the tables and figures, as defined by their orbit (semi-major axis and eccentricity), albedo, and thermal characteristics.
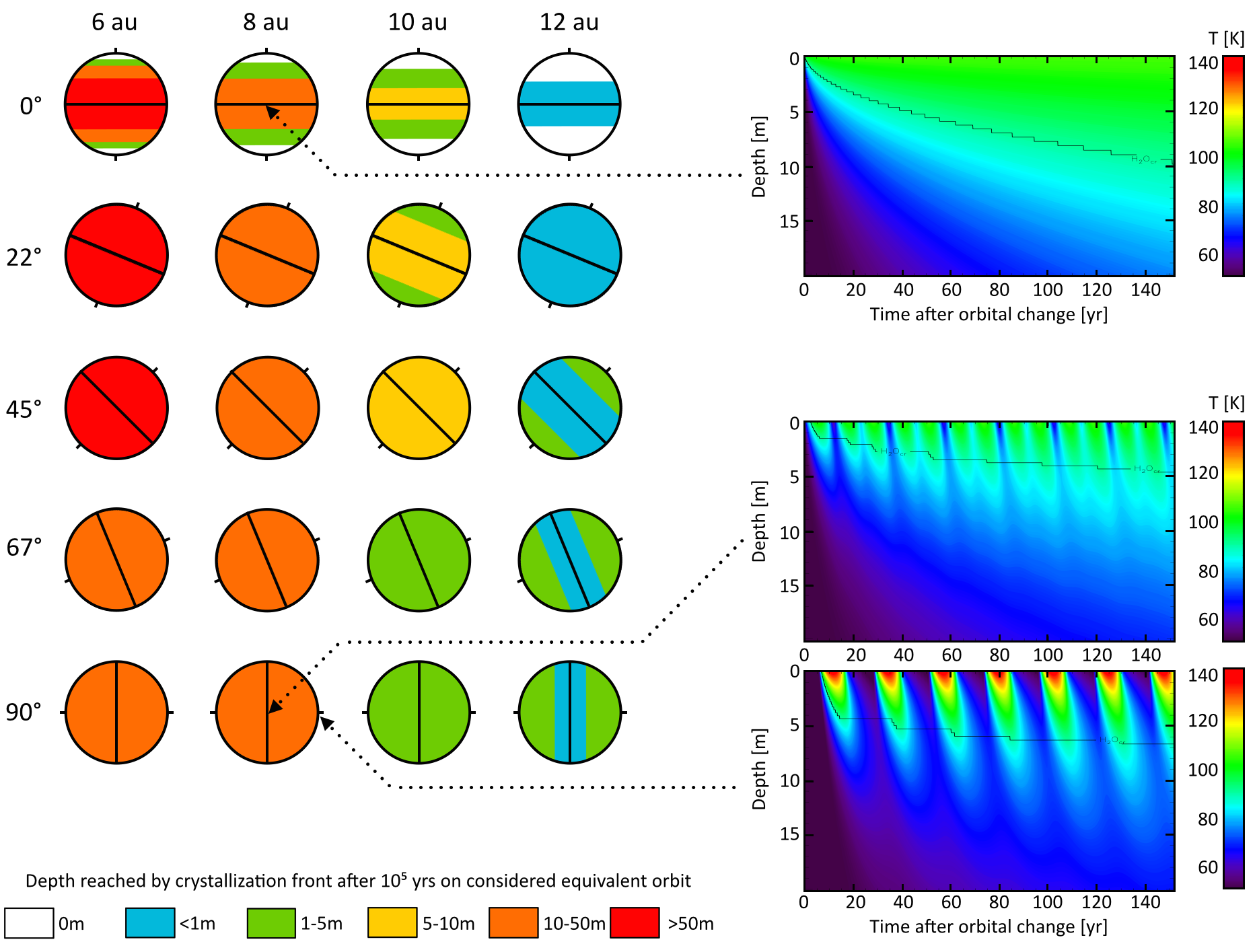
On the left, the figure shows the depths where the crystallisation front could have stopped for different orbital configurations (columns show the heliocentric distance, row correspond to various obliquities, i.e. the tilt of the spin axis with respect to the orbital plane), after 100,000 years of evolution in the giant planet region (typical for Centaurs). On the right, the figure shows how the heat wave and crystallisation front progress in the subsurface layer for three cases (at 8 au for all, and from top to bottom, under the equator at 0° obliquity, under the equator at 90° obliquity and under the pole at 90° obliquity), during the time the objects may be active (few hundred years at this distance, after an orbital change labelled time = 0). The black lines show the crystallisation fronts. From Snodgrass et al. (2017), adapted from Guilbert- Lepoutre (2012).
The main outputs of my study are the following:
- Crystallization can be triggered even at heliocentric distances as large as 16 au, especially if objects have a high obliquity, so it can indeed by a source of activity for Centaurs. Amorphous water ice can nonetheless survive even at the surface of Centaurs, especially beyond 10 au.
- Because it would be accompanied by the release of trapped volatiles, the transition from amorphous to crystalline water ice is an efficient source of outgassing, especially for small heliocentric distances and small obliquities. Cometary activity due to crystallization can be expected up to 10–12 au, which is broadly consistent with the observations of active Centaurs.
- However, as the crystallization front propagates deeper and deeper inside Centaurs, cometary activity may stop. This means that outgassing due to the crystallization of amorphous ice can only be sustained for a limited amount of time, hundreds to thousands of years. This implies that should crystallization be the driver for the activity of Centaurs, these Centaurs should have suffered from a recent change in their orbital parameters.
For now, there hasn’t been any observation to contradict either Jewitt (2009) or Guilbert-Lepoutre (2012). The limitation, though, remains that amorphous water ice hasn’t been directly detected yet, so there is not direct confirmation of these studies either. I hope that future observations, perhaps with JWST, as well as the modeling of some new phase transitions, will help to shed lights on the origin of active Centaurs.
NIRSpec GTO website
A quick news: the JWST/NIRSpec website about GTO programs is now live here. I put below the details for my program, also available on the ESA site.
This program will focus on constraining the surface composition of 2 Charon-like objects, i.e. mid-sized Transneptunian objects (TNOs) and in particular looking for possible presence of ammonia at their surface. The recent observations of the NASA/New Horizons probe have shown that Charon’s surface is possibly indicative of past cryovolcanic activity. Ammonia and ammonia hydrates, common antifreezes, have been detected in the spectra obtained both from the probe and ground-based facilities. We have selected 2 targets, Orcus and 2003 AZ84, with physical properties similar to Charon, for which past cryovolcanism could have been an interesting evolutionary path. Traces of volatiles such as ammonia and/or hydrates have been found in ground-based spectra of Orcus. We will further investigate this possibility, and open a new window into constraining the inventory of volatiles at the surface of Orcus by observing also the 3-5micron range, where fundamental absorption bands of C-H-, N-H- and O-H-bearing ices can be found. Recent occultation results suggest that 2003 AZ84 may have a canyon-like feature on its surface, similar to the large canyon seen on the surface of Charon. Given its other physical characteristics, this object is selected as our second target. Ground-based observations of this fainter TNO suggest that crystalline water ice may be the dominant volatile species present at its surface, though the presence of minor species cannot be constrained.
Note that as the launch approaches, I’ll be publishing some information on this program and general help with JWST tools specific to Solar System observations, so stay tuned!
More on JWST, more on NIRSpec, also here and there.
ISSI Team on Main Belt Comets
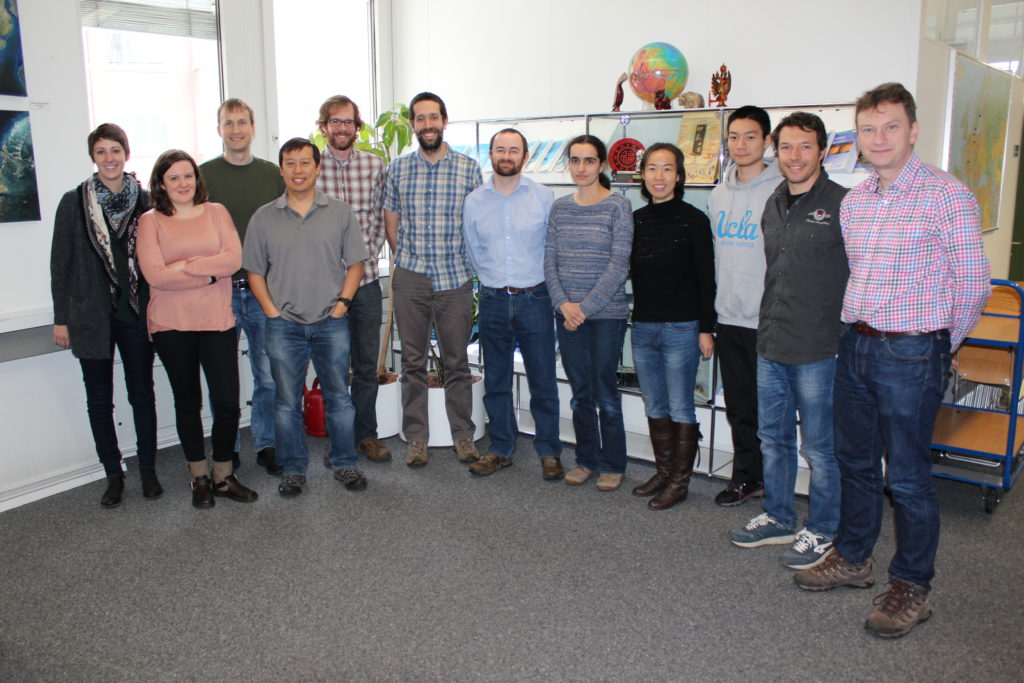
This week, the ISSI team led by Colin Snodgrass was meeting in wintery Bern, Switzerland. This has been an intense and fruitful week discussing the current state of our understanding of Main Belt Comets, and in particular of the detection of water (either gas or ice) in the Main Belt of Asteroids.
For the past 10 years or so, comet-like objects – i.e. objects which loose dusty material providing them with a coma and/or tail – have been discovered on asteroid-like orbits. Many physical processes can actually explain why and how asteroids could loose material from their surface/subsurface, which do not involve the sublimation of water ice like for comets (see the very good reviews by Heny Hsieh or David Jewitt). However, some of these objects do show a repeted activity, observed when they go through perihelion, on multiple orbits. This seems like an indication that water ice is indeed sublimating, like for regular comets. The issue is that no water has ever been detected for any of these objects. So is it because we cannot see it, due to technical limitations which could potentially be lifted with upcoming facilites like ALMA or JWST, or is this telling us something fundamental about the properties and evolution of these objects? We’ve agreed on one thing for sure, we need an other meeting!
Spectral mapping of comet 67P – DPS/EPSC 2016
This year, the two major international conferences for planteray science – DPS and EPSC – are joined in Pasadena, CA. Obviously, I’m excited to see the great results from the three space missions currently investigating small bodies: ESA/Rosetta, NASA/New Horizons and NASA/Dawn. Here is my contribution to the Rosetta results, with a spectral mapping in visible and near-infrared made from the ground with ESO/VLT instruments MUSE and SINFONI. The observations (made in March 2016) were great, but the comet was really at the limit of what we could achieve from the ground, even if it seemed to have a small outburst just before we started observing in Chile.
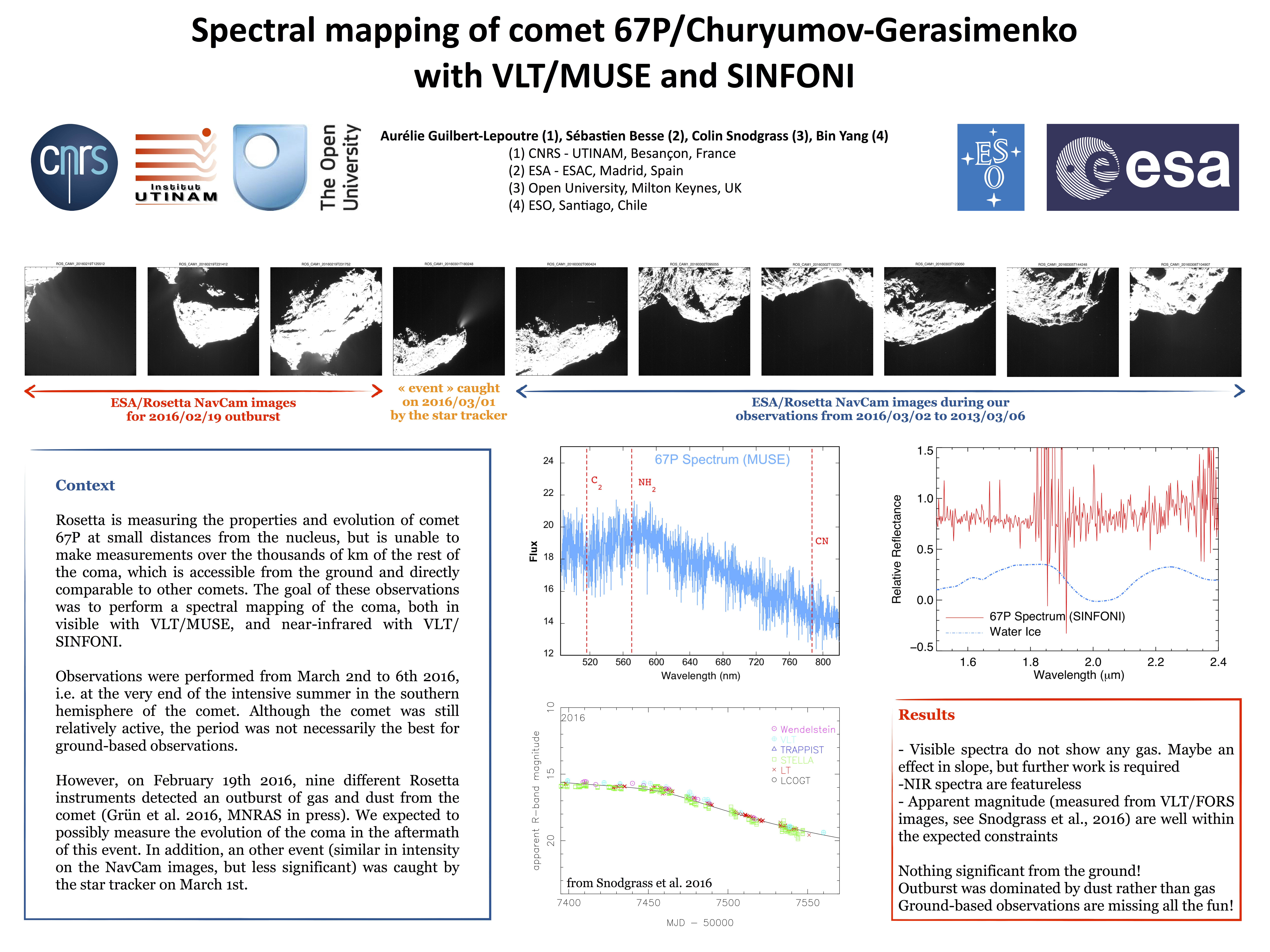
Thermal processing of 67P – ESLAB 2016
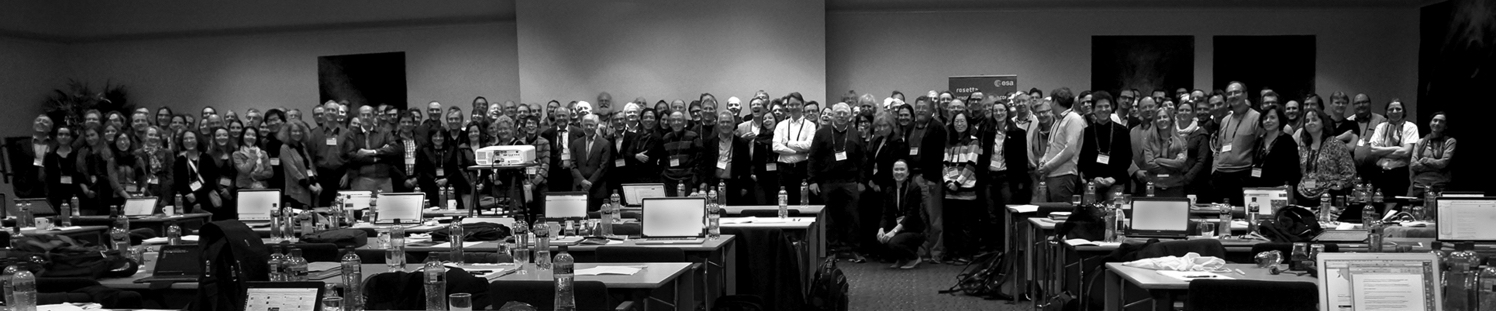
Credits: Ekatarina Smirnova – http://www.ekaterina-smirnova.com/50th-eslab/
From Giotto to Rosetta. The 50th ESLAB symposium was focussed on 30 years of cometary science, triggered by these two impressive space missions. I’ve been invited to talk about the evolution of comets, a subject at the heart of debate in the community. This lead to a contribution in the MNRAS special issue “From Giotto to Rosetta”, written in collaboration with my colleagues from Israel. In this article, we review the most relevant studies, their results, and their implications for the study of 67P/Churyumov-Gerasimenko, the target of Rosetta.
The studies we have reviewed in the paper hold a strong statistical significance when it comes to interpreting the current results of the Rosetta mission, even if those studies were not designed to be applied to 67P in the first place. Indeed, using the current thermo-physical properties of 67P as initial parameters for studying its possible past processing would defeat the purpose of demonstrating that the physical processes responsible for such processing did actually shape the nucleus as observed today. Rather, the current thermo-physical parameters of 67P should be outputs of these models, and initial parameters should be derived by a trial and error procedure which would involve a significant and almost impossible work, given that the orbital evolution of 67P (hence the thermal processing linked to its orbital evolution) cannot be traced back accurately for more than hundreds of years.
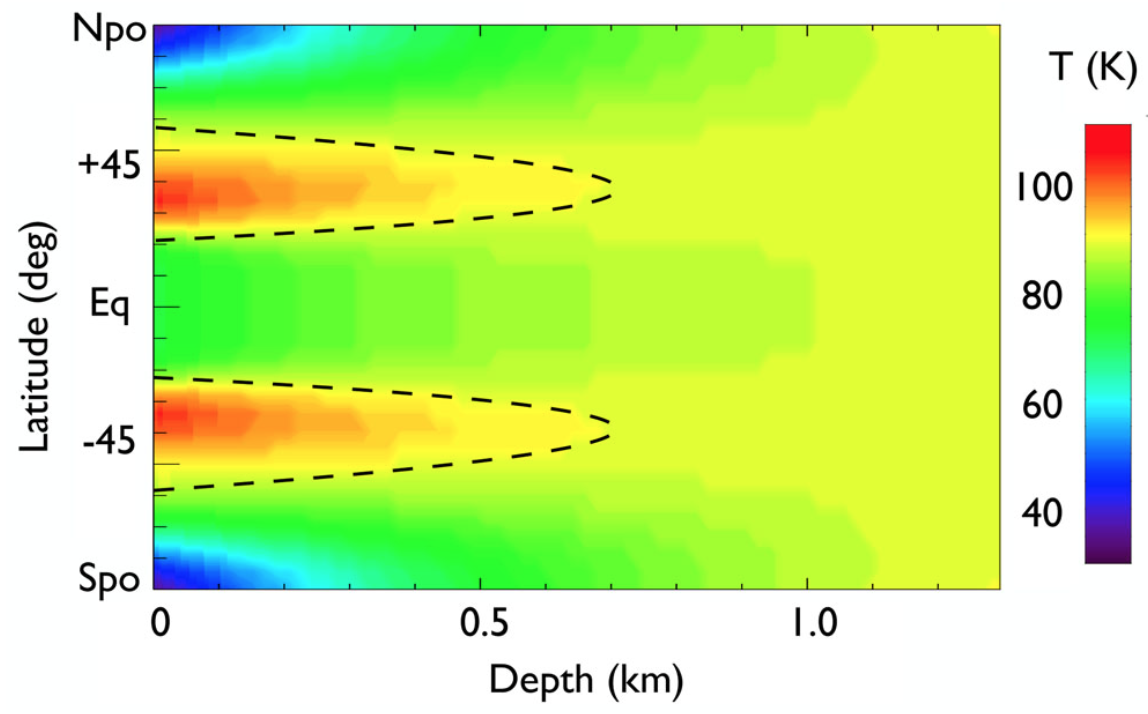
Temperature distribution along a meridian, for a comet with a non-uniform surface albedo. A non-uniform temperature distribution at the surface triggers the development of non-uniform internal compositions, since temperature-dependent phase transitions like sublimation or crystallization proceed at different rates in the subsurface. Lateral heat fluxes are very inefficient at erasing such heterogeneous structures: they are therefore long-lived. From Guilbert-Lepoutre et al. (2016), adapted from Guilbert-Lepoutre & Jewitt (2011).
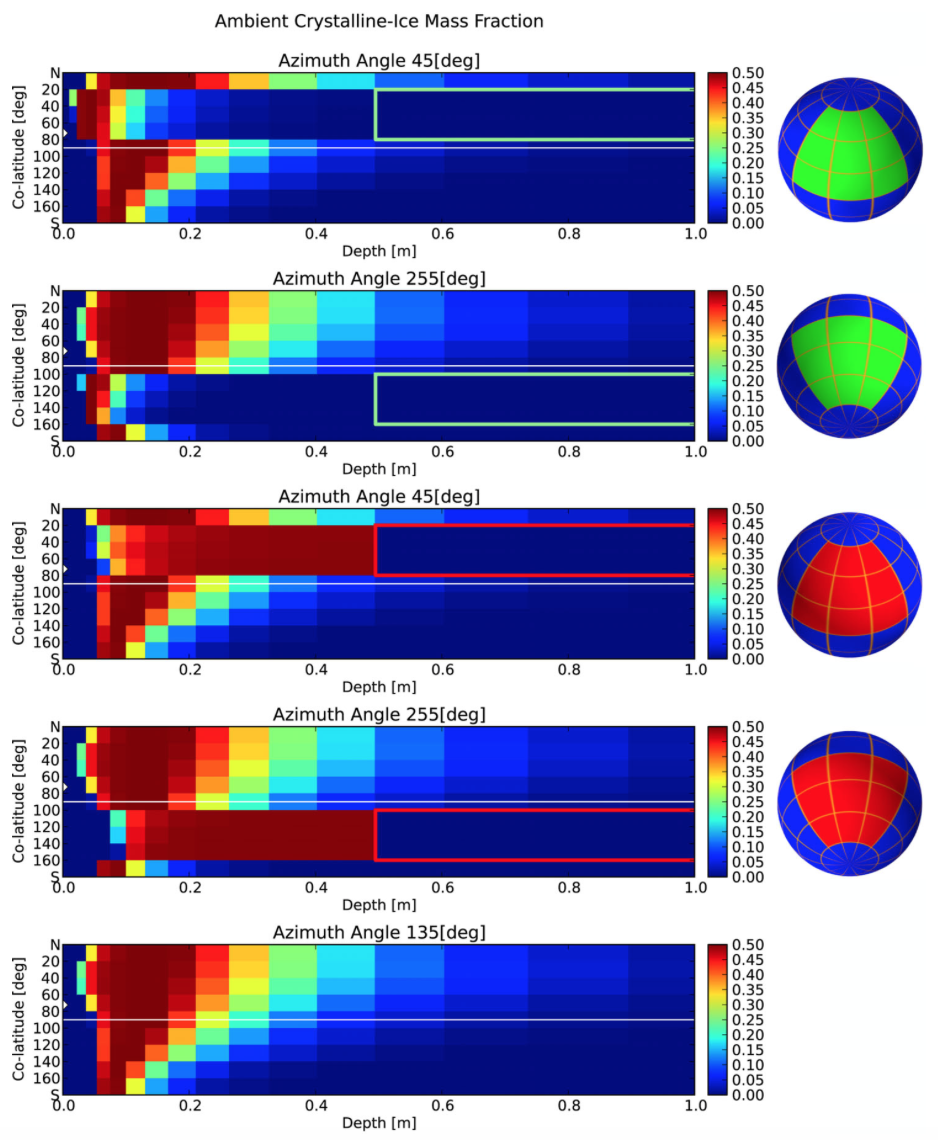
Mass fraction of crystalline ice as a function of depth, down to 1m (abscissa) and co-latitude (ordinate). Each panel represents a “slice” of the nucleus. The bottom panel is used as the homogeneous reference case. The red and green frames mark the location of patches: red – porous ice, green – compact dust. The position of each patch is illustrated on the right-hand side of each panel. From Guilbert-Lepoutre et al. (2016), adapted from Rosenberg & Prialnik (2010).
First, the article deals with how the long-term thermal processing of comets can lead to layered internal structures. This process – which I’ll call radial differentiation – is probably the most accepted within the community, although the debate remains as to how significant (= how deep) the effects are… For this process, the actual orbital evolution does play a significant role, since the final stratigraphy does depend on how comet nuclei are injected into the inner solar system. And that is still a big unknown today. While the inner part of a comet nucleus may remain unaltered by early evolution, its outer layers are expected to be processed, perhaps to a significant degree. In a way, the outermost layers protect the deep ones, especially in the presence of a dust mantle, which is an efficient insulator.
We decided to also address the issue of lateral differentiation, studied by Guilbert-Lepoutre & Jewitt (2011). Intuitively, we indeed expect that phase transitions like sublimation or amorphous water ice crystallization (at the heart of cometary activity) would proceed at different rates inside the nucleus if the energy balance varies over the surface of a comet: this is illustrated by the figure on the left. Are these two processes effectively observed on 67P? Is the layering due to thermal processing? We wished to stress that far from claiming that evolution produced such heterogeneity, the inevitable past processing of the nucleus cannot be overlooked when interpreting the complex results of observations provided by Rosetta, especially since the properties of 67P’s subsurface on a smaller scale are consistent with thermal processing playing an active part in shaping the current nucleus characteristics.
Ultimately, we needed to address the effects on internal inhomogenetities on the activity of a comet, whether these inhomogeneities are primordial or due to evolution, as studied by Rosenberg & Prialnik (2011). To summarize, the main effect of an initially inhomogeneous configuration, where patches are randomly distributed throughout the outer part of the nucleus (see figure on the left), is a somewhat erratic activity, with outbursts at all heliocentric distances. They would affect water and other volatiles production rates, local erosion, the progression of crystallization, the dust mantle thickness, produce activity on the night side, a differentiation of dust grain size in the surface layer, and an uneven surface by differential erosion, creating mountains, depressions and terraces. One should keep in mind that simplified models such as described here, are not meant to reproduce the behaviour of a given comet, but rather help understand the general behaviour patterns of comet nuclei and the diversity of surface structures in itself. With that in mind, many arguments have been put forward already to suggest that the nucleus of 67P is heterogeneous: the outbursts observed by Rosetta, and some of the geological features, may thus be the consequence of such heterogeneities.
Finally, we addressed the issue raised by the presence of circular depressions at the surface of 67P. They offer the exciting prospect of looking into the internal structure and the thermo-physical processing of comet nuclei. We performed new calculations, and found that it is very unlikely that 100–200 m depressions were formed on 67P’s current orbit, since none of the known phase transitions is able to proceed sufficiently fast and deep to open a cavity that would then collapse, or to directly excavate the superficial layers. Therefore, the presence of circular depressions can be regarded as some evidence for the past processing of the nucleus. Since the results from the orbital evolution model indicate that the previous orbits would have been even less likely to produce these features (having perihelia distances further from the Sun than this current one), it may indeed be the case that circular depressions on 67P are the result of a long-term processing that still awaits to be understood.
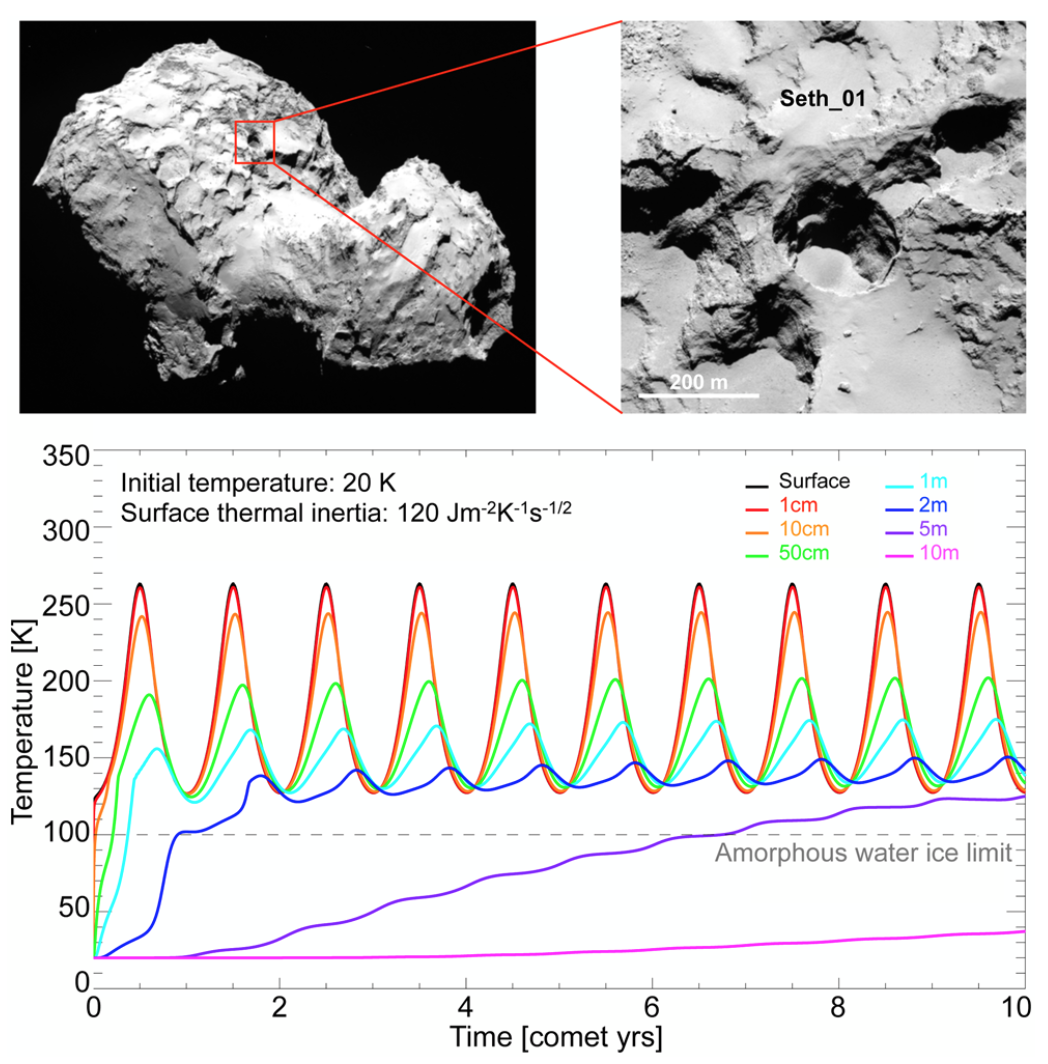
Bottom panel shows the evolution of the temperature at different depths for illumination conditions similar to those of the Seth_01 circular depression shown above. One comet year is 6.44 yr. From Guilbert-Lepoutre et al. (2016).
Could water ice have survived in Jupiter Trojans? – COSPAR 2015
The 2nd edition of the COSPAR symposium is focussed on “Water and Life in the Universe”. A perfect occasion for me to present my work on Jupiter Trojans, which was published last year on Icarus (here). For this study, I was interested in understanding whether water ice, had it been incorporated in Jupiter Trojans when they were formed, could have survived until today.
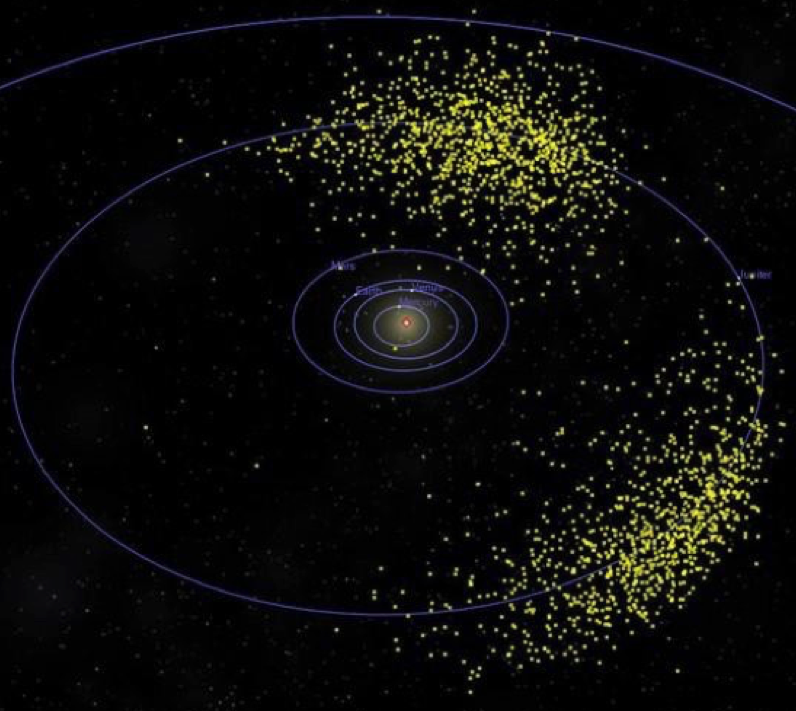
Jupiter Trojans are a very interesting population of small bodies: they are asteroids dynamically locked into the L4 and L5 Lagrangian points of the Jupiter-Sun system. They are basically sharing Jupiter’s orbit, and have been following the planet since they’ve been “put” there. Their origins is still debated though: they could be remnants of the population of planetesimals that formed Jupiter itself, and thus tell us a lot on the material incorporated in the giant planet. Or they could have been formed much further away from the Sun, and were trapped in the Lagrangian points during their chaotic orbital evolution as described by the Nice model. It’s reasonable to think that it is a combination of both.
When it comes to their physical properties, Jupiter Trojans do look like dead or dormant comets in many aspects: they share similar albedos, colors, spectral features… Even their low density suggests that they could be ice-rich objects. All the information we have gathered so far are consistent with Jupiter Trojans having volatile-rich interiors, and surfaces made of a porous dusty crust, which may or may not have been formed by past sublimation (although to this day no cometary activity has ever been detected for any Jupiter Trojan). However, given the possibility that Trojans may have been formed at large heliocentric distance in ice-rich regions, it is important to understant if this ice could have survive, so that we could use this property as a constrain for dynamical models of the evolution of the solar system.
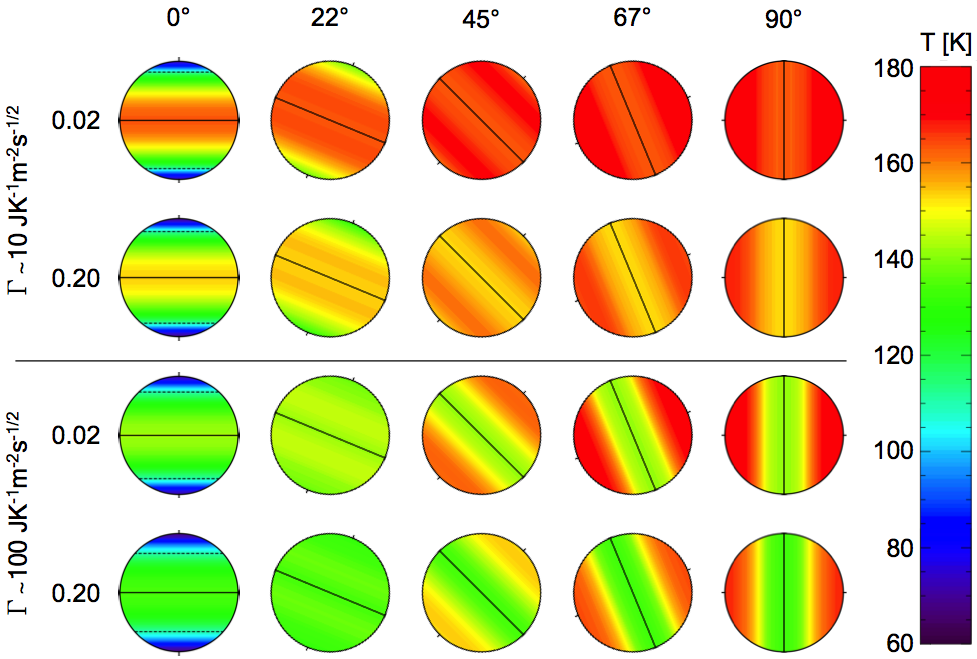
Temperatures at the surface of Trojans, in the ideal case of spherical bodies. See text on the right for more information. From Guilbert-Lepoutre (2014).
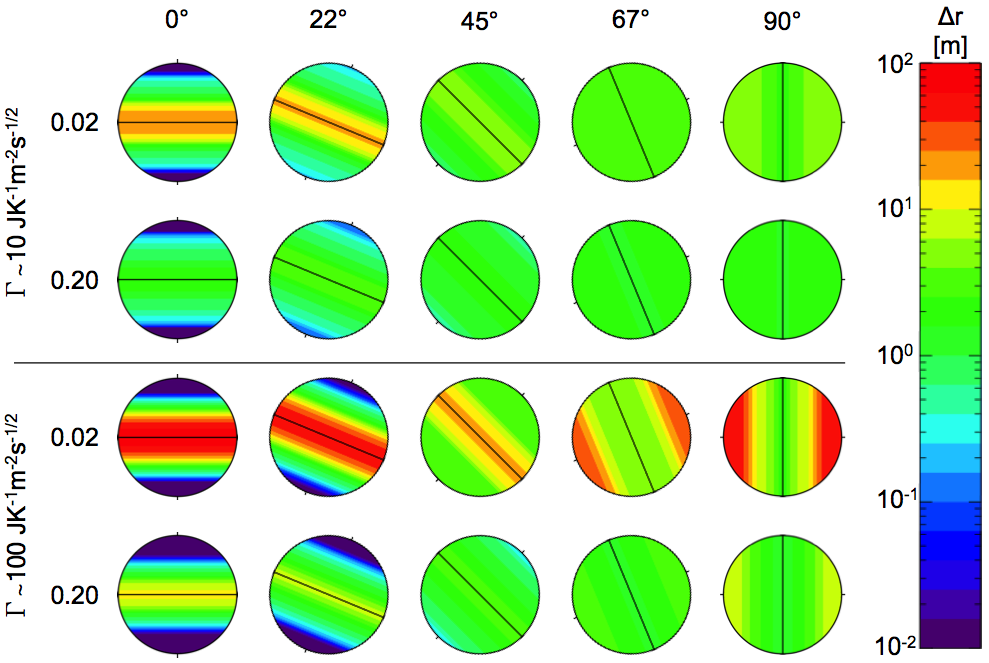
Depth at which water ice could be found, under a “blanket” of porous dust. See text on the right for more information. From Guilbert-Lepoutre (2014).
The first figure on the left shows the temperature at the surface of Trojans for various obliquities (the tilt of the spin axis with respect to the orbital plane – 0° = poles are never illuminated, 90° = poles are facing the Sun at perihelion/aphelion), two albedos which encompass those measured for Trojans (2% and 20%), and two values of the thermal inertia (which governs how the heat is transfered to the subsurface). First, it is important to notice that ice could have been able to survive at the surface of these objects for low values of the obliquity, as its was actually observed after this work got published by Brown et al. In addition, these are “ideal” temperatures computed for spherical bodies, and therefore do not account for the actual shapes of Trojans which can create large areas of shadows at the surface. It is thus definitely possible for water ice to survive at the surface of Trojans, in areas of low illumination…
Now, in areas where ice cannot survive at the surface – which remains most of the cases I’ve studied – we need to know how deep ice could be, and whether we’d have any chance to access it, with radar observations for instance. The depth at which water ice can be found is shown on the second figure on the left, again for various obliquities, albedos and thermal inertias. This figure is very green, which is a very good news since it means that it could be found within the first meter in most cases.
In the paper, I’ve also shown that the depth at which water ice can be found varies with the dynamical scenario, i.e. whether Trojans never came closer than 5 au, or they followed Jupiter when it migrated inward for example. Therefore, the water ice depth could be used as a tracer of the past orbital evolution of these objects, and inform us on the dynamical evolution of the solar system. However, keep in mind that these are the results of computations from a model with numerous free or poorly constrained parameters, so the exact depths found in the article should not be taken as face values, but rather as order of magnitudes – they all remain within the reach of in situ radar investigations.
Modeling the evolution of comets
Welcome to this new webpage, where I hope I’ll be able to share some of my research! Articles published in peer-reviewed journals can be found here. I have two main areas of expertise: observations of small bodies, and modeling of their thermo-physical evolution. Let’s talk about the latter. Indeed, my recent paper “On the evolution of comets” has just been published. It started with a workshop I had organized while I was still working at ESA: Surface-Interior Interactions on Small Bodies. Back in 2006, some colleagues had been writing a book, part of the ISSI collection: Heat and Gas Diffusion in Comet Nuclei (Huebner et al. 2006). The book is dressing a big picture of thermal evolution modeling after the Giotto mission, and before Rosetta (it remains my bible). Now our idea was to assess where thermal modeling had been heading since that book had been written, what remained to be understood, and how the Rosetta mission (which would start to give results in the coming months at that time) would perhaps be able to address our questions. After lots of interesting discussions, we decided to write a review paper for the Space Science Reviews. I’d like to summarize here the discussion, so to explain why studying the thermal evolution of comets is relevant and important.
We study comets because we believe that they hold invaluable clues on the formation and evolution of our planetary system. In comparison to planets, they have undergone much less alteration, having been exposed to fewer evolutionary processes that acted preferentially at their surface. Therefore, they should have retained a relatively pristine record of the conditions prevailing during the early phases of the solar system. However, we still have not been able to determine which of the observed physical, chemical and orbital characteristics of comets will provide the best clues to their origin, after they have evolved for more than 4 Gyr in a time-varying radiative and collisional environment. Comet nuclei could be formed of a single component, either relatively uniform in composition, or heterogeneous if comets accreted material from different regions when migrating through the protoplanetary disk. Alternatively, comet nuclei may be made of multiple components loosely bound together. These sub-units may similarly be relatively uniform, or could originate from different regions of the protoplanetary disk. Furthermore, some of the observed nuclei might be collisional debris of larger, possibly chemically differentiated parent bodies. The subsequent evolution of comet nuclei involves some processing from radiogenic heating, space weathering and large- and small-scale collisions, which could have modified their primordial structures and compositions to various degrees. When comets enter the inner solar system and become active, they start to loose mass at a very high rate. The effects of activity on comet nuclei involve a layering of the composition, a substantial non-even erosion and modification of their size and shape, and may eventually result in the death of comets.
From an orbital point of view, it is clear that the evolution of comets is dominated by chaos. Ultimately, it is entirely possible that the evolutionary track may be specific to each comet, which would complicate the task of constraining their origins as a whole population. Because evolutionary processes may lead to so many different outcomes, we need to focus on their long-lasting effects, with statistical significance. But before we are able to track back the origins of comet nuclei, we have identified two issues that do need to be addressed before we can make any progress: how does activity actually work? what is the role of collisions?