The 2nd edition of the COSPAR symposium is focussed on “Water and Life in the Universe”. A perfect occasion for me to present my work on Jupiter Trojans, which was published last year on Icarus (here). For this study, I was interested in understanding whether water ice, had it been incorporated in Jupiter Trojans when they were formed, could have survived until today.
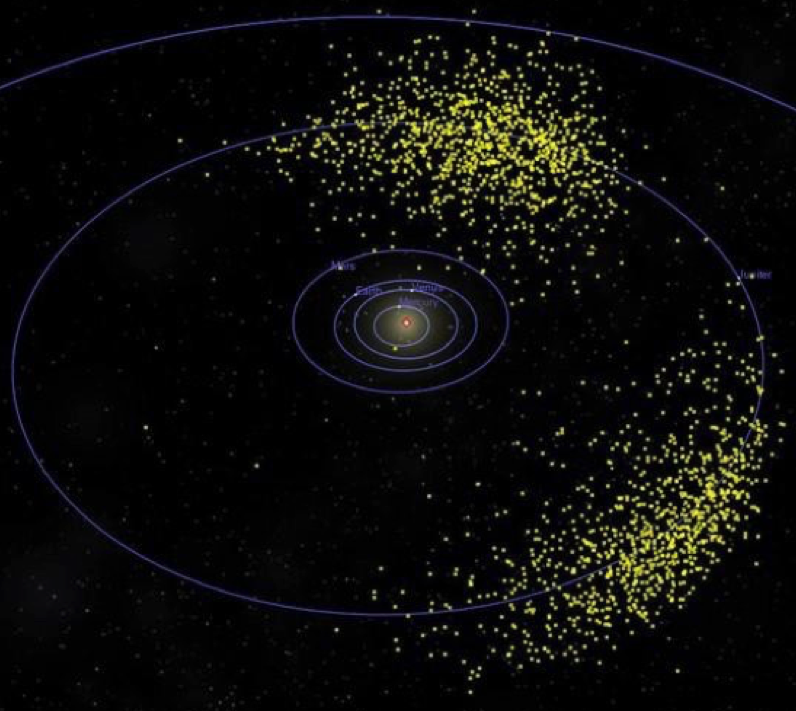
Jupiter Trojans are a very interesting population of small bodies: they are asteroids dynamically locked into the L4 and L5 Lagrangian points of the Jupiter-Sun system. They are basically sharing Jupiter’s orbit, and have been following the planet since they’ve been “put” there. Their origins is still debated though: they could be remnants of the population of planetesimals that formed Jupiter itself, and thus tell us a lot on the material incorporated in the giant planet. Or they could have been formed much further away from the Sun, and were trapped in the Lagrangian points during their chaotic orbital evolution as described by the Nice model. It’s reasonable to think that it is a combination of both.
When it comes to their physical properties, Jupiter Trojans do look like dead or dormant comets in many aspects: they share similar albedos, colors, spectral features… Even their low density suggests that they could be ice-rich objects. All the information we have gathered so far are consistent with Jupiter Trojans having volatile-rich interiors, and surfaces made of a porous dusty crust, which may or may not have been formed by past sublimation (although to this day no cometary activity has ever been detected for any Jupiter Trojan). However, given the possibility that Trojans may have been formed at large heliocentric distance in ice-rich regions, it is important to understant if this ice could have survive, so that we could use this property as a constrain for dynamical models of the evolution of the solar system.
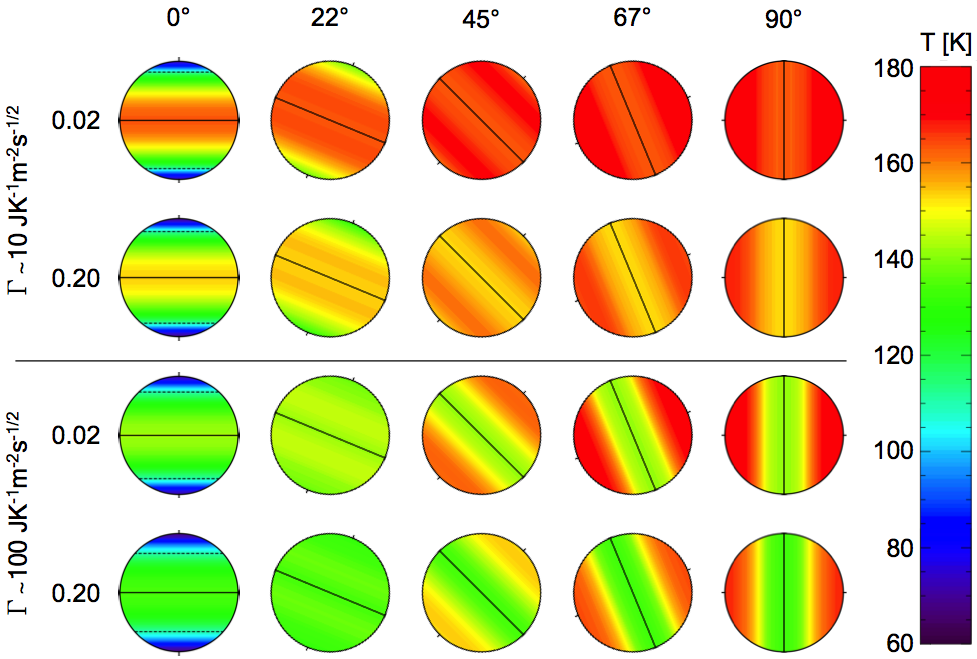
Temperatures at the surface of Trojans, in the ideal case of spherical bodies. See text on the right for more information. From Guilbert-Lepoutre (2014).
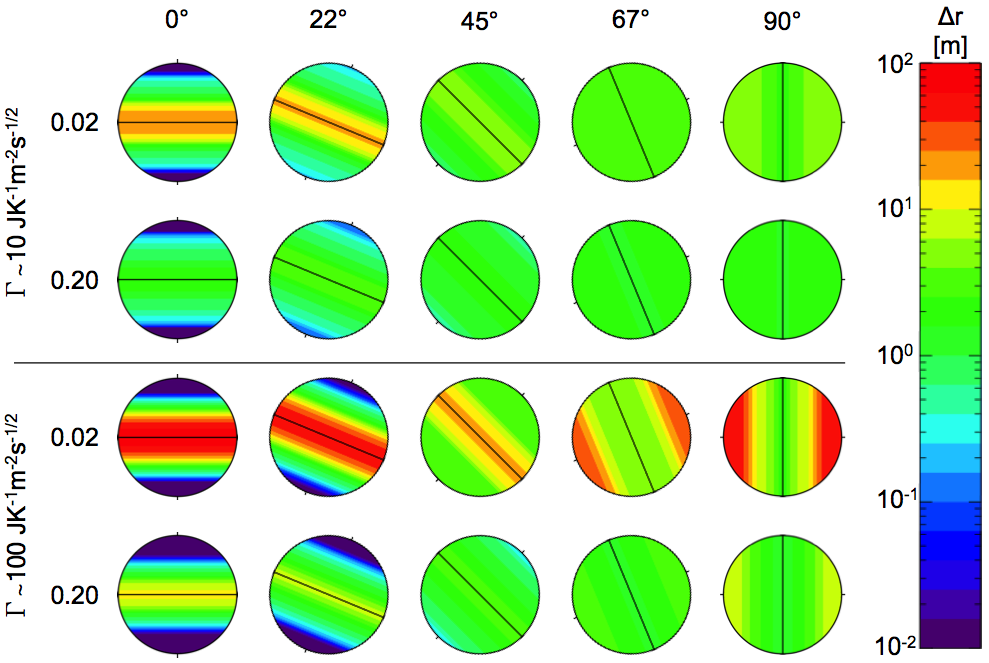
Depth at which water ice could be found, under a “blanket” of porous dust. See text on the right for more information. From Guilbert-Lepoutre (2014).
The first figure on the left shows the temperature at the surface of Trojans for various obliquities (the tilt of the spin axis with respect to the orbital plane – 0° = poles are never illuminated, 90° = poles are facing the Sun at perihelion/aphelion), two albedos which encompass those measured for Trojans (2% and 20%), and two values of the thermal inertia (which governs how the heat is transfered to the subsurface). First, it is important to notice that ice could have been able to survive at the surface of these objects for low values of the obliquity, as its was actually observed after this work got published by Brown et al. In addition, these are “ideal” temperatures computed for spherical bodies, and therefore do not account for the actual shapes of Trojans which can create large areas of shadows at the surface. It is thus definitely possible for water ice to survive at the surface of Trojans, in areas of low illumination…
Now, in areas where ice cannot survive at the surface – which remains most of the cases I’ve studied – we need to know how deep ice could be, and whether we’d have any chance to access it, with radar observations for instance. The depth at which water ice can be found is shown on the second figure on the left, again for various obliquities, albedos and thermal inertias. This figure is very green, which is a very good news since it means that it could be found within the first meter in most cases.
In the paper, I’ve also shown that the depth at which water ice can be found varies with the dynamical scenario, i.e. whether Trojans never came closer than 5 au, or they followed Jupiter when it migrated inward for example. Therefore, the water ice depth could be used as a tracer of the past orbital evolution of these objects, and inform us on the dynamical evolution of the solar system. However, keep in mind that these are the results of computations from a model with numerous free or poorly constrained parameters, so the exact depths found in the article should not be taken as face values, but rather as order of magnitudes – they all remain within the reach of in situ radar investigations.